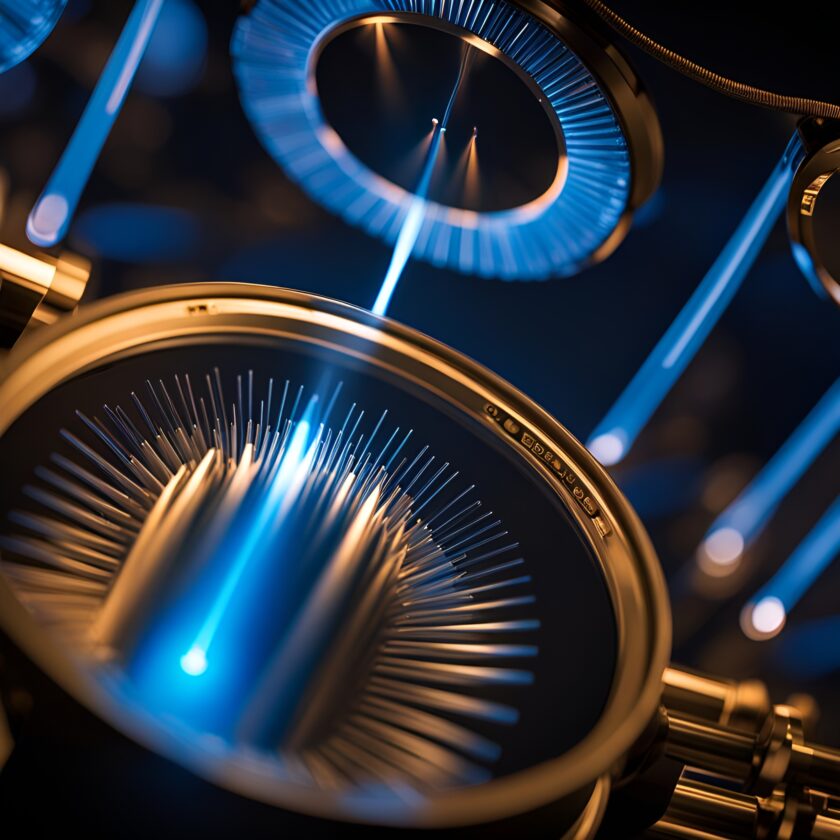
“A quantum engine in the BCS-BEC transition.” This was the title of a study published last September in the academic journal “Nature.” The research was conducted by a team of Japanese and German researchers working within the Quantum Systems division of the Okinawa Institute of Science and Technology. The term “quantum engine” implies the development of a system capable of converting the energy associated with the quantum states of a group of atoms or subatomic particles into usable macroscopic work by altering their interaction modes.
Understanding the meaning of this definition can be challenging for those not familiar with the field. It requires knowledge of the principles of quantum physics, which are extremely complex and necessitate a radical shift in how we perceive matter. However, to provide an idea of the study’s findings, one can think of processes occurring in a traditional propulsion system, such as a thermal engine. It is known that specific combustion phenomena generate the energy that crankshafts convert into driving force. Through calibrated heat emissions defining isobaric transformations at constant pressure in the ideal Diesel cycle and isochoric transformations at constant volume in the ideal Otto cycle.
Expansion and compression but no combustion
The quantum engine operates in a similar manner, but without the need for air/fuel mixtures and without combustion. Instead, the phases of expansion and compression of the working gas remain. These are defined by adiabatic transformations, allowing the possibility of utilizing mechanisms designed to convert the succession of these processes into mechanical energy. Replacing combustion phenomena is a state transition of the particles composing the working fluid. A transition from “Bose-Einstein condensate” to “Fermi gas” capable of increasing energy according to the “Pauli exclusion principle.”
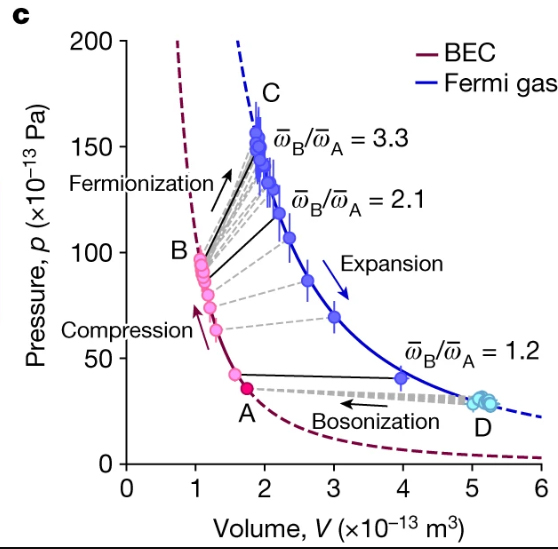
And here a couple of explanations are needed. The first one concerns quantum statistics applied to the ensemble of lithium atoms used in the processes investigated by the research team. Quantum statistics, in simple terms, refers to that branch of statistics that examines the behavior of subatomic particles based on the laws of quantum mechanics that determine their interaction. There are two main quantum statistics. The “Fermi-Dirac” statistics applies to all “fermions,” particles that have half-integer spin, an intrinsic property of every particle related to its angular momentum.
“Fermions” and “bosons”
Electrons, protons, and neutrons, for example, are fermions. These particles adhere to the aforementioned “Pauli exclusion principle,” formulated by physicist Wolfgang Pauli in 1925. It states that two fermions cannot occupy the same quantum state at the same time. This fundamental principle helps understand the stability of atoms and the interaction of individual particles. Such as the orderly distribution of electrons around a nucleus, excluding possible overlaps in the same quantum spaces.
The second statistics, Bose-Einstein statistics, applies instead to “bosons.” These are particles with spin described by an integer number and do not adhere to the “Pauli exclusion principle.”
This characteristic allows particles to share the same quantum states with others, creating the state of matter defined as “unusual,”. Predicted as early as 1924 by scientists Satyendra Nath Bose and Albert Einstein. In “Bose-Einstein condensates,” a large number of particles, typically ultracold atoms, meaning close to absolute zero in terms of temperature, occupy the same fundamental quantum state in a kind of collective “supercell” with unique properties.
All elementary particles mediating fundamental forces, such as photons of electromagnetism, are bosons. Although the described statistics apply to different particles, in 1957 scientists John Bardeen, Leon Cooper, and Robert Schrieffer developed a theory that described the possibility of fermions behaving based on Bose-Einstein statistics, as bosons. The “BCS theory,” named after the initials of the three scientists’ surnames, which earned them the Nobel Prize in 1972, explains some quantum effects, including superconductivity. It also states that fermions with half-integer spin can associate in pairs, known as “Cooper pairs.”
At its core, the Pauli exclusion principle
Behaving like bosons with integer spin and occupying the same quantum state in a condensed form. Hence the title of the research “a quantum engine in the BCS-BEC transition,” mentioned at the beginning of the article and at the core of the Pauli engine’s operation. Scientists started with a “Bose-Einstein molecular condensate,” confined in a “harmonic trap” defined by the overlap of a magnetic field and a laser beam. Starting from this state, the working cycle first involves compressing the condensate by increasing the power of the laser acting on the gas. Then, by increasing the intensity of the magnetic field, the condensate returns to its original state. An increase in energy is caused by the “Pauli exclusion principle.”
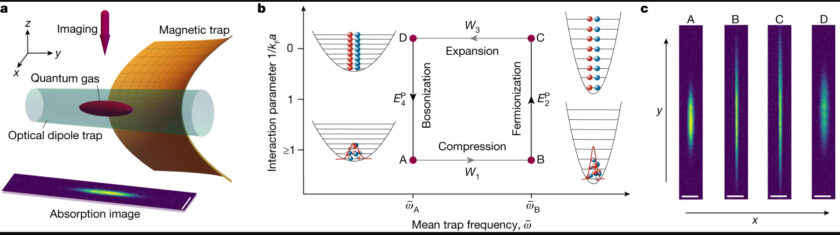
B) Pauli engine cycle. Starting from a Bose-Einstein molecular condensate, point “A,” increasing the radial oscillation frequency of the particles by adjusting the power of the confinement lasers performs work on the system, “W1,” resulting in gas compression from “A” to “B.” The transition to fermionic gas, from “B” to “C,” is achieved by increasing the intensity of the magnetic field, causing an increase in energy due to the Pauli exclusion principle, which prevents two fermions from occupying the same quantum state. This leads to a rearrangement of the particles. Expansion from “C” to “D” is achieved by adjusting the lasers again and reducing their power as well as the oscillation frequency of the particles. Finally, the system is returned to a condensed state, from “D” to “A,” by reducing the intensity of the magnetic field.
C) Spectroscopic images of the phases of the cycle. The expansion of the gas from “B” to “C” due to the increase in Pauli energy is evident. Source: Nature
Quantum states and superpositions
In practice, what happens is that the particles initially in superposition return to occupy different quantum states, resulting in a rearrangement of the system. This transition essentially corresponds to the combustion phase of a thermal engine, where chemical energy is converted into heat. At this point, by reducing the power of the laser, gas expansion is achieved, allowing the system to perform work. The final step is the return to the condensed state by again acting on the magnetic field and once again excluding the “Pauli principle.”
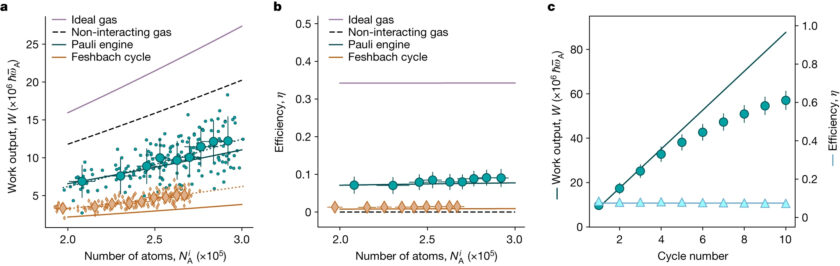
The difference in energy defined in the “BEC-BCS” transition, and vice versa, is fundamental to the engine’s operation. Allowing for the expansion and compression of the gas that results in mechanical work. It’s worth noting that, currently, all effects produced have been achieved experimentally on a sample of gas targeted in the research titled “a quantum engine in the BCS-BEC transition.” By measuring the work produced in the expansion phase and comparing it with the energy supplied to the system to carry out the transitions, scientists have calculated an efficiency of 25 percent. The path to applying the process to a propulsion system is therefore still long and will also need to overcome several functional gaps, not least of which is maintaining gases at temperatures close to absolute zero to limit energy absorption.
Quantum engine
Author: Jacopo Oldani
Translated by ChatGPT